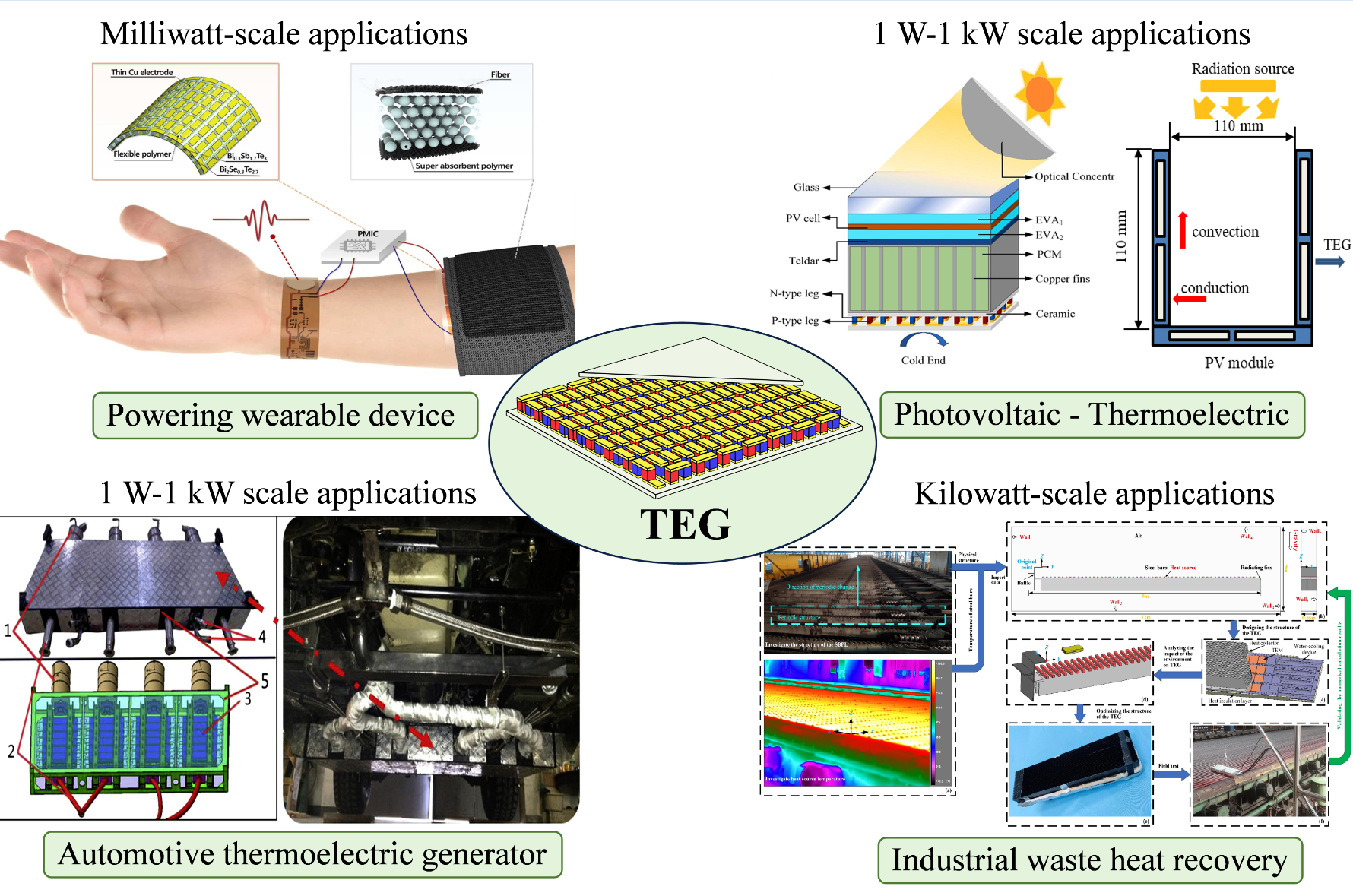
Downloads
Download


This work is licensed under a Creative Commons Attribution 4.0 International License.
Review
A Comprehensive Review of Thermoelectric Generators from Micropower Supply to Kilowatt System
Shuo Yang, Hao Chen and Ding Luo *
Shaanxi Key Laboratory of New Transportation Energy and Automotive Energy Saving, Chang’an University,
Xi’an 710064, China
* Correspondence: Ding_L@outlook.com
Received: 14 March 2025; Revised: 9 April 2025; Accepted: 10 April 2025; Published: 22 April 2025
Abstract: Energy crisis and carbon emissions are two increasingly prominent issues in our society. As one of the clean energy sources, thermoelectric power generation is a promising alternative energy technology to convert heat into electricity. As long as there is a heat source, thermoelectric generators can provide electricity for watches, sensors, electronics, spacecraft, etc., and can also be used to recover waste heat, such as automobile exhaust heat, industrial waste heat, ship waste heat, etc. This study proposes a novel classification paradigm based on power output (microwatt, 1 W–1 kW, >1 kW), systematically revealing the technological characteristics at each power level: the microwatt level relies on flexible materials and compatibility with human body heat, while the kilowatt level requires the integration of high-temperature materials and optimized thermal management. The study also demonstrates that performance can be significantly enhanced through asymmetric geometric designs and non-equilibrium synthesis processes. This work provides a comprehensive design framework, from material innovation to large-scale integration, for next-generation thermoelectric systems, addressing the theoretical gap in techno-economic analysis.
Keywords:
thermoelectric generators waste heat recovery kilowatt thermal managementReferences
- Ge, M.; Li, Z.; Wang, Y.; et al. Experimental study on thermoelectric power generation based on cryogenic liquid cold energy. Energy 2021, 220, 119746. https://doi.org/10.1016/j.energy.2020.119746.
- Luo, D.; Yu, Y.; Yan, Y.; et al. Increasing power densities in a thermoelectric generator by stacking and incorporating dual heat pipes. Device 2024, 2, 100435. https://doi.org/10.1016/j.device.2024.100435.
- Luo, D.; Liu, Z.; Cao, J.; et al. Feasibility and parametric study of a groove-type thermoelectric generator under multiphysics field conditions. Appl. Therm. Eng. 2025, 259, 124972. https://doi.org/10.1016/j.applthermaleng.2024.124972.
- Luo, D.; Wu, Z.; Zhang, Z.; et al. Transient thermal analysis of a thermoelectric-based battery thermal management system at high temperatures. Energy 2025, 318, 134833. https://doi.org/10.1016/j.energy.2025.134833.
- He, T.; Nair, S.K.; Babu, P.; et al. A novel conceptual design of hydrate based desalination (HyDesal) process by utilizing LNG cold energy. Appl. Energy 2018, 222, 13–24. https://doi.org/10.1016/j.apenergy.2018.04.006.
- Shih, W.-C.; Matsuda, M.; Konno, K.; et al. Tailored thermoelectric performance of poly(phenylene butadiynylene)s/carbon nanotubes nanocomposites towards wearable thermoelectric generator application. Compos. Part B Eng. 2024, 286, 111779. https://doi.org/10.1016/j.compositesb.2024.111779.
- Bennett, G. Space Nuclear Power: Opening the Final Frontier. In Proceedings of the 4th International Energy Conversion Engineering Conference and Exhibit (IECEC), San Diego, CA, USA, 26–29 June 2006.
- Kraemer, D.; Jie, Q.; McEnaney, K.; et al. Concentrating solar thermoelectric generators with a peak efficiency of 7.4%. Nat. Energy 2016, 1, 16153. https://doi.org/10.1038/nenergy.2016.153.
- Zhao, R.; Zhu, N.; Zhao, X.; et al. Multi-objective optimization of a novel photovoltaic-thermoelectric generator system based on hybrid enhanced algorithm. Energy 2025, 319, 135046. https://doi.org/10.1016/j.energy.2025.135046.
- Suresh Prasanna, C.; Harish, S.; Archana, J.; et al. Interfacial energy barrier tuning in MnO2/MoS2/Carbon fabric integrated with low resistance textrode for highly efficient wearable thermoelectric generator. Carbon 2024, 218, 118609. https://doi.org/10.1016/j.carbon.2023.118609.
- Luo, D.; Li, Z.; Yang, S.; et al. Improved performance of the thermoelectric generator by combining vapor chambers and circular fins. Energy 2025, 320, 135354. https://doi.org/10.1016/j.energy.2025.135354.
- Luo, D.; Yang, S.; Li, Z.; et al. Transient energy, exergy, and economic analysis of an automotive thermoelectric generator with different structures. Appl. Energy 2025, 377, 124494. https://doi.org/10.1016/j.apenergy.2024.124494.
- Luo, D.; Yang, S.; Zhang, H.; et al. Performance improvement of an automotive thermoelectric generator by introducing a novel split fin structure. Appl. Energy 2025, 382, 125218. https://doi.org/10.1016/j.apenergy.2024.125218.
- Yang, S.; Chen, H.; Yang, X.; et al. Design optimization of split fins in heat pipe-based thermoelectric generators. Energy 2025, 322, 135547. https://doi.org/10.1016/j.energy.2025.135547.
- Luo, Q.; Li, P.; Cai, L.; et al. A Thermoelectric Waste-Heat-Recovery System for Portland Cement Rotary Kilns. J. Electron. Mater. 2015, 44, 1750–1762. https://doi.org/10.1007/s11664-014-3543-1.
- Nour Eddine, A.; Chalet, D.; Faure, X.; et al. Optimization and characterization of a thermoelectric generator prototype for marine engine application. Energy 2018, 143, 682–695. https://doi.org/10.1016/j.energy.2017.11.018.
- Miao, Z.; Meng, X.; Li, X. Design a high-performance thermoelectric generator by analyzing industrial heat transfer. Appl. Energy 2023, 347, 121403. https://doi.org/10.1016/j.apenergy.2023.121403.
- Liu, X.; Wang, K.; Shen, Z. A novel strategy of inserting radiation shields to enhance the performance of thermoelectric generator systems for industrial high-temperature heat recovery. Energy 2024, 301, 131704. https://doi.org/10.1016/j.energy.2024.131704.
- Luo, D.; Wang, R.C. Experimental Test and Estimation of the Equivalent Thermoelectric Properties for a Thermoelectric Module. J. Energy Resour. Technol.-Trans. Asme 2021, 143, 122102. https://doi.org/10.1115/1.4050132.
- Luo, D.; Chen, H.; Chen, W.-H.; et al. Interdependent optimization strategies for material, module, and system designs in thermoelectric devices. Device 2025, 100752. https://doi.org/10.1016/j.device.2025.100752.
- Bell, L.E. Cooling, Heating, Generating Power, and Recovering Waste Heat with Thermoelectric Systems. Science 2008, 321, 1457. https://doi.org/10.1126/science.1158899.
- Twaha, S.; Zhu, J.; Yan, Y.; et al. A comprehensive review of thermoelectric technology: Materials, applications, modelling and performance improvement. Renew. Sustain. Energy Rev. 2016, 65, 698–726. https://doi.org/10.1016/j.rser.2016.07.034.
- Zheng, X.F.; Liu, C.X.; Yan, Y.Y.; et al. A review of thermoelectrics research—Recent developments and potentials for sustainable and renewable energy applications. Renew. Sustain. Energy Rev. 2014, 32, 486–503. https://doi.org/10.1016/j.rser.2013.12.053.
- Luo, D.; Wang, R.; Yu, W.; et al. Parametric study of a thermoelectric module used for both power generation and cooling. Renew. Energy 2020, 154, 542–552. https://doi.org/10.1016/j.renene.2020.03.045.
- Wu, Y.; Ma, W.; Guo, Z.-Y. Governing equations of thermoelectric generators. Int. J. Heat Mass Transf. 2025, 241, 126737. https://doi.org/10.1016/j.ijheatmasstransfer.2025.126737.
- Luo, D.; Wang, R.; Yu, W. Comparison and parametric study of two theoretical modeling approaches based on an air-to-water thermoelectric generator system. J. Power Sources 2019, 439, 227069. https://doi.org/10.1016/j.jpowsour.2019.227069.
- Siddique, A.R.M.; Mahmud, S.; Heyst, B.V. A review of the state of the science on wearable thermoelectric power generators (TEGs) and their existing challenges. Renew. Sustain. Energy Rev. 2017, 73, 730–744. https://doi.org/10.1016/j.rser.2017.01.177.
- Ma, Y.; Hao, Q.; Poudel, B.; et al. Enhanced Thermoelectric Figure-of-Merit in p-Type Nanostructured Bismuth Antimony Tellurium Alloys Made from Elemental Chunks. Nano Lett. 2008, 8, 2580–2584. https://doi.org/10.1021/nl8009928.
- Ohta, M.; Biswas, K.; Lo, S.-H.; et al. Enhancement of Thermoelectric Figure of Merit by the Insertion of MgTe Nanostructures in p-type PbTe Doped with Na2Te. Adv. Energy Mater. 2012, 2, 1117–1123. https://doi.org/10.1002/aenm.201100756.
- Joshi, G.; Lee, H.; Lan, Y.; et al. Enhanced Thermoelectric Figure-of-Merit in Nanostructured p-type Silicon Germanium Bulk Alloys. Nano Lett. 2008, 8, 4670–4674. https://doi.org/10.1021/nl8026795.
- Kim, S.I.; Lee, K.H.; Mun, H.A.; et al. Dense dislocation arrays embedded in grain boundaries for high-performance bulk thermoelectrics. Science 2015, 348, 109–114. https://doi.org/10.1126/science.aaa4166.
- Wang, Y.; Liu, W.-D.; Shi, X.-L.; et al. Enhanced thermoelectric properties of nanostructured n-type Bi2Te3 by suppressing Te vacancy through non-equilibrium fast reaction. Chem. Eng. J. 2020, 391, 123513. https://doi.org/10.1016/j.cej.2019.123513.
- Meroz, O.; Elkabets, N.; Gelbstein, Y. Enhanced Thermoelectric Properties of n-Type Bi2Te3–xSex Alloys following Melt-Spinning. ACS Appl. Energy Mater. 2020, 3, 2090–2095. https://doi.org/10.1021/acsaem.9b02133.
- Zheng, Y.; Liu, C.; Miao, L.; et al. Extraordinary thermoelectric performance in MgAgSb alloy with ultralow thermal conductivity. Nano Energy 2019, 59, 311–320. https://doi.org/10.1016/j.nanoen.2019.02.045.
- Wu, Y.; Chen, Z.; Nan, P.; et al. Lattice Strain Advances Thermoelectrics. Joule 2019, 3, 1276–1288. https://doi.org/10.1016/j.joule.2019.02.008.
- Rogl, G.; Grytsiv, A.; Rogl, P.; et al. n-Type skutterudites (R,Ba,Yb)yCo4Sb12 (R = Sr, La, Mm, DD, SrMm, SrDD) approaching ZT ≈ 2.0. Acta Mater. 2014, 63, 30–43. https://doi.org/10.1016/j.actamat.2013.09.039.
- Tsai, Y.-F.; Wei, P.-C.; Chang, L.; et al. Compositional Fluctuations Locked by Athermal Transformation Yielding High Thermoelectric Performance in GeTe. Adv. Mater. 2021, 33, 2005612. https://doi.org/10.1002/adma.202005612.
- Saiga, Y.; Du, B.; Deng, S.K.; et al. Thermoelectric properties of type-VIII clathrate Ba8Ga16Sn30 doped with Cu. J. Alloys Compd. 2012, 537, 303–307. https://doi.org/10.1016/j.jallcom.2012.05.049.
- Shi, X.; Zhao, T.; Zhang, X.; et al. Extraordinary n-Type Mg3SbBi Thermoelectrics Enabled by Yttrium Doping. Adv. Mater. 2019, 31, 1903387. https://doi.org/10.1002/adma.201903387.
- Luo, D.; Liu, Z.; Cao, J.; et al. Performance investigation and optimization of an L-type thermoelectric generator. Energy 2024, 307, 132768. https://doi.org/10.1016/j.energy.2024.132768.
- Wang, R.; Meng, Z.; Luo, D.; et al. A Comprehensive Study on X-Type Thermoelectric Generator Modules. J. Electron. Mater. 2020, 49, 4343–4354. https://doi.org/10.1007/s11664-020-08152-4.
- Luo, D.; Zhang, H.; Cao, J.; et al. Innovative design of an annular thermoelectric generator for enhanced automotive waste heat recovery. Energy Convers. Manag. 2024, 313, 118584. https://doi.org/10.1016/j.enconman.2024.118584.
- de Oca, O.Y.E.-M.; Olivares-Robles, M.A. Dynamic performance optimization of two-stage thermoelectric generator: Impact of different geometric leg shapes in each stage. Energy Rep. 2024, 11, 597–610. https://doi.org/10.1016/j.egyr.2023.12.032.
- Bian, M.; Xu, Z.; Tang, X.; et al. Tri-objective and multi-parameter geometric optimization of two-stage radioisotope thermoelectric generator based on NSGA-II. Appl. Therm. Eng. 2025, 258, 124685. https://doi.org/10.1016/j.applthermaleng.2024.124685.
- Zhang, Q.; Liao, J.; Tang, Y.; et al. Realizing a thermoelectric conversion efficiency of 12% in bismuth telluride/skutterudite segmented modules through full-parameter optimization and energy-loss minimized integration. Energy Environ. Sci. 2017, 10, 956–963. https://doi.org/10.1039/C7EE00447H.
- He, H.; Xie, Y.; Zuo, Q.; et al. Optimization analysis for thermoelectric performance improvement of biconical segmented annular thermoelectric generator. Energy 2024, 306, 132397. https://doi.org/10.1016/j.energy.2024.132397.
- Sun, Y.; Zhai, P.; Wang, S.; et al. Performance enhancement of segmented annular thermoelectric generator based on multi-parameter and multi-objective optimization. Therm. Sci. Eng. Prog. 2024, 47, 102245. https://doi.org/10.1016/j.tsep.2023.102245.
- Nozariasbmarz, A.; Suarez, F.; Dycus, J.H.; et al. Thermoelectric generators for wearable body heat harvesting: Material and device concurrent optimization. Nano Energy 2020, 67, 104265. https://doi.org/10.1016/j.nanoen.2019.104265.
- Hyland, M.; Hunter, H.; Liu, J.; et al. Wearable thermoelectric generators for human body heat harvesting. Appl. Energy 2016, 182, 518–524. https://doi.org/10.1016/j.apenergy.2016.08.150.
- Van Toan, N.; Thi Kim Tuoi, T.; Van Hieu, N.; et al. Thermoelectric generator with a high integration density for portable and wearable self-powered electronic devices. Energy Convers. Manag. 2021, 245, 114571. https://doi.org/10.1016/j.enconman.2021.114571.
- Van Bavel, M.; Leonov, V.; Yazicioglu, R.F.; et al. Wearable battery-free wireless 2-channel EEG systems powerd by energy scavengers. Sens. Transducers J. 2008, 94.
- Lv, H.; Liang, L.; Zhang, Y.; et al. A flexible spring-shaped architecture with optimized thermal design for wearable thermoelectric energy harvesting. Nano Energy 2021, 88, 106260. https://doi.org/10.1016/j.nanoen.2021.106260.
- Kim, C.S.; Yang, H.M.; Lee, J.; et al. Self-Powered Wearable Electrocardiography Using a Wearable Thermoelectric Power Generator. ACS Energy Lett. 2018, 3, 501–507. https://doi.org/10.1021/acsenergylett.7b01237.
- Beltrán-Pitarch, B.; García-Cañadas, J. A novel vacuum pressure sensor using a thermoelectric device. Vacuum 2020, 172, 109088. https://doi.org/10.1016/j.vacuum.2019.109088.
- Kim, Y.J.; Gu, H.M.; Kim, C.S.; et al. High-performance self-powered wireless sensor node driven by a flexible thermoelectric generator. Energy 2018, 162, 526–533. https://doi.org/10.1016/j.energy.2018.08.064.
- Guan, M.; Wang, K.; Xu, D.; et al. Design and experimental investigation of a low-voltage thermoelectric energy harvesting system for wireless sensor nodes. Energy Convers. Manag. 2017, 138, 30–37. https://doi.org/10.1016/j.enconman.2017.01.049.
- Champier, D. Thermoelectric generators: A review of applications. Energy Convers. Manag. 2017, 140, 167–181. https://doi.org/10.1016/j.enconman.2017.02.070.
- Shittu, S.; Li, G.; Xuan, Q.; et al. Electrical and mechanical analysis of a segmented solar thermoelectric generator under non-uniform heat flux. Energy 2020, 199, 117433. https://doi.org/10.1016/j.energy.2020.117433.
- Cotfas, D.T.; Enesca, A.; Cotfas, P.A. Enhancing the performance of the solar thermoelectric generator in unconcentrated and concentrated light. Renew. Energy 2024, 221, 119831. https://doi.org/10.1016/j.renene.2023.119831.
- Cao, Z.; Li, W. A day-night solar thermoelectric generator enabled by phase change material and forced water cooling. Sol. Energy 2024, 268, 112315. https://doi.org/10.1016/j.solener.2024.112315.
- Luo, D.; Wang, R.; Yu, W.; et al. Modelling and simulation study of a converging thermoelectric generator for engine waste heat recovery. Appl. Therm. Eng. 2019, 153, 837–847. https://doi.org/10.1016/j.applthermaleng.2019.03.060.
- Yang, W.; Jin, C.; Zhu, W.; et al. Taguchi optimization and thermoelectrical analysis of a pin fin annular thermoelectric generator for automotive waste heat recovery. Renew. Energy 2024, 220, 119628. https://doi.org/10.1016/j.renene.2023.119628.
- Luo, D.; Li, Z.; Yan, Y.; et al. Design and optimization of a thermoelectric generator with dimple fins to achieve higher net power. Appl. Therm. Eng. 2024, 252, 123735. https://doi.org/10.1016/j.applthermaleng.2024.123735.
- Ge, M.; Zhao, C.; Xiao, Y.; et al. Experimental study on enhancing thermoelectric performance of exhaust thermoelectric generator using multi-orifice plate. J. Clean. Prod. 2025, 486, 144446. https://doi.org/10.1016/j.jclepro.2024.144446.
- Luo, D.; Yang, S.; Yan, Y.; et al. Performance improvement of the automotive thermoelectric generator by extending the hot side area of the heat exchanger through heat pipes. Energy Convers. Manag. 2024, 310, 118472. https://doi.org/10.1016/j.enconman.2024.118472.
- Pacheco, N.; Brito, F.P.; Vieira, R.; et al. Compact automotive thermoelectric generator with embedded heat pipes for thermal control. Energy 2020, 197, 117154. https://doi.org/10.1016/j.energy.2020.117154.
- Liu, X.; Deng, Y.D.; Li, Z.; et al. Performance analysis of a waste heat recovery thermoelectric generation system for automotive application. Energy Convers. Manag. 2015, 90, 121–127. https://doi.org/10.1016/j.enconman.2014.11.015.
- Zhang, Y.; Cleary, M.; Wang, X.; et al. High-temperature and high-power-density nanostructured thermoelectric generator for automotive waste heat recovery. Energy Convers. Manag. 2015, 105, 946–950. https://doi.org/10.1016/j.enconman.2015.08.051.
- Georgopoulou, C.A.; Dimopoulos, G.G.; Kakalis, N.M.P. A modular dynamic mathematical model of thermoelectric elements for marine applications. Energy 2016, 94, 13–28. https://doi.org/10.1016/j.energy.2015.10.130.
- Kuroki, T.; Kabeya, K.; Makino, K.; et al. Thermoelectric Generation Using Waste Heat in Steel Works. J. Electron. Mater. 2014, 43, 2405–2410. https://doi.org/10.1007/s11664-014-3094-5.