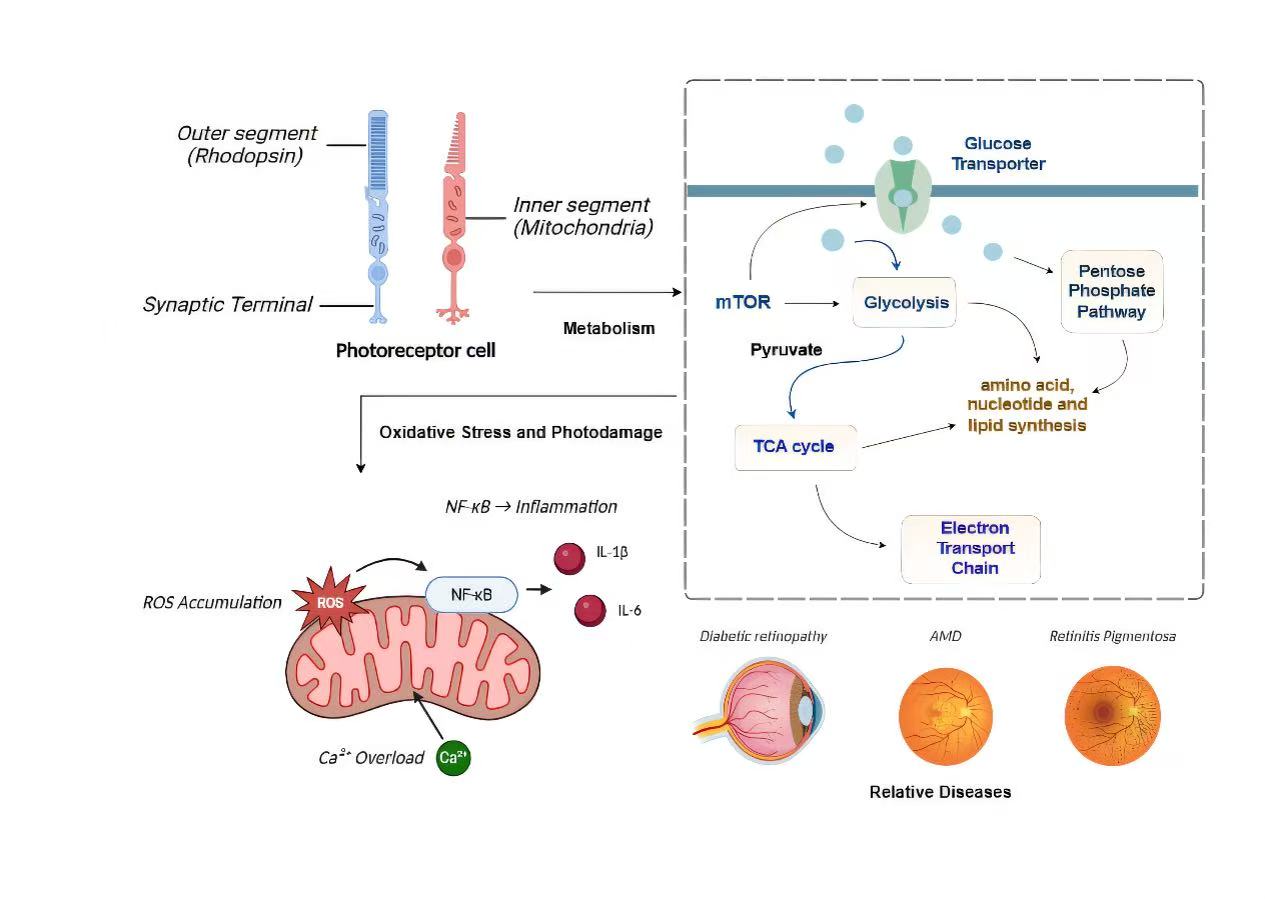
Downloads
Download


This work is licensed under a Creative Commons Attribution 4.0 International License.
Review
The Metabolic Underpinnings of Retinal Health and Disease: A Focus on Photoreceptor Cells
Jianbo Jin 1,2, Qiuping Liu 3,*, and Cheng Li 1,2,4,*
1 Eye Institute & Affiliated Xiamen Eye Center, School of Medicine, Xiamen University, Xiamen 361102, China
2 Fujian Provincial Key Laboratory of Ophthalmology and Visual Science, Xiamen 361102, China
3 Department of Ophthalmology, The First Affiliated Hospital of University of South China, Hengyang Medical School, University of South China, Hengyang 421001, China
4 Shen Zhen Research Institute, Xiamen University, Shenzhen 518057, China
* Correspondence: liuqiuping1983@gmail.com (Q.L.); cheng-li@xmu.edu.cn (C.L.)
Received: 27 October 2024; Revised: 9 December 2024; Accepted: 19 February 2025; Published: 14 April 2025
Abstract: The photoreceptor cells of the retina, encompassing rods and cones, are pivotal in the transduction of light signals into chemical ones, thereby initiating the visual process. This review explores the metabolic bedrock of these cells, which are not only crucial for preserving retinal health but are also susceptible to metabolic stress, precipitating a spectrum of retinal pathologies. The manuscript elucidates the intricate metabolic processes of photoreceptor cells, the synergistic metabolic interplay between photoreceptors and the retinal pigment epithelium (RPE), and appraises the metabolic shifts within photoreceptor cells under conditions of photic injury and diverse pathological states, as well as the ramifications of these metabolic perturbations for retinal function. The review culminates with a prospective horizon scan of research in photoreceptor cell metabolism, charting anticipated investigative trajectories.
Keywords:
photoreceptor cells retinal metabolism retinal diseasesReferences
- Altshuler, D.M. Specification of cell type in the vertebrate retina. Dev. Vis. Syst. 1991, 37, 139–143.
- Wetts, R.; Fraser, S.E. Multipotent precursors can give rise to all major cell types in the frog retina. Science 1988, 239, 1142–1145.
- Turner, D.L.; Snyder, E.Y.; Cepko, C.L. Lineage-independent determination of cell type in the embryonic mouse retina. Neuron 1990, 4, 833–845.
- Hofmann, K.P.; Lamb, T.D. Rhodopsin, light-sensor of vision. Prog. Retin. Eye Res. 2023, 93, 101116. https://doi.org/10.1016/j.preteyeres.2022.101116.
- Nickells, R.W. The First Steps in Seeing. Arch. Ophthalmol. 1999, 117, 550.
- Xu, J.; Zhao, C.; Kang, Y. The Formation and Renewal of Photoreceptor Outer Segments. Cells 2024, 13, 1357. https://doi.org/10.3390/cells13161357.
- Lamb, T.D. Photoreceptor physiology and evolution: Cellular and molecular basis of rod and cone phototransduction. J. Physiol. 2022, 600, 4585–4601. https://doi.org/10.1113/jp282058.
- Turner, D.L.; Cepko, C.L. A common progenitor for neurons and glia persists in rat retina late in development. Nature 1987, 328, 131–136.
- Arendt, D. Evolution of eyes and photoreceptor cell types. Int. J. Dev. Biol. 2003, 47, 563.
- Hanna, J.; David, L.A.; Touahri, Y.; Fleming, T.; Screaton, R.A.; Schuurmans, C. Beyond Genetics: The Role of Metabolism in Photoreceptor Survival, Development and Repair. Front. Cell Dev. Biol. 2022, 10, 887764. https://doi.org/10.3389/fcell.2022.887764.
- Morrow, E.M.; Furukawa, T.; Cepko, C.L. Vertebrate photoreceptor cell development and disease. Trends Cell Biol. 1998, 8, 353–358.
- Carter-Dawson, L.D.; Lavail, M.M. Rods and cones in the mouse retina. II. Autoradiographic analysis of cell generation using tritiated thymidine. J. Comp. Neurol. 1979, 188, 263–272.
- Holt, C.E.; Bertsch, T.W.; Ellis, H.M.; Harris, W.A. Cellular determination in the Xenopus retina is independent of lineage and birth date. Neuron 1988, 1, 15–26.
- Rapaport, D.H.; Wong, L.L.; Wood, E.D.; Yasumura, D.; LaVail, M.M. Timing and topography of cell genesis in the rat retina. J. Comp. Neurol. 2004, 474, 304–324. https://doi.org/10.1002/cne.20134.
- Morrow, E.M.; Belliveau, M.J.; Cepko, C.L. Two Phases of Rod Photoreceptor Differentiation during Rat Retinal Development. J. Neurosci. 1998, 18, 3738–3748.
- Swaroop, A.; Kim, D.; Forrest, D. Transcriptional regulation of photoreceptor development and homeostasis in the mammalian retina. Nat. Rev. Neurosci. 2010, 11, 563–576.
- Adler, R.; Hatlee, M. Plasticity and differentiation of embryonic retinal cells after terminal mitosis. Science 1989, 243, 391–393.
- Cayouette, M.; Poggi, L.; Harris, W.A. Lineage in the vertebrate retina. Trends Neurosci. 2006, 29, 563–570.
- Agathocleous, M.; Harris, W.A. From progenitors to differentiated cells in the vertebrate retina. Annu. Rev. Cell Dev. Biol. 2009, 25, 45–69. https://doi.org/10.1146/annurev.cellbio.042308.113259.
- Brodrick, E.; Jékely, G. Photobehaviours guided by simple photoreceptor systems. Anim. Cogn. 2023, 26, 1817–1835. https://doi.org/10.1007/s10071-023-01818-6.
- Joyal, J.S.; Gantner, M.L.; Leh, S. Retinal energy demands control vascular supply of the retina in development and disease: The role of neuronal lipid and glucose metabolism. Prog. Retin. Eye Res. 2018, 64, 131–156.
- Wang, Y.; Patti, G.J. The Warburg effect: A signature of mitochondrial overload. Trends Cell Biol. 2023, 33, 1014–1020. https://doi.org/10.1016/j.tcb.2023.03.013.
- Barba, I.; Carrillo-Bosch, L.; Seoane, J. Targeting the Warburg Effect in Cancer: Where Do We Stand? Int. J. Mol. Sci. 2024, 25, 3142. https://doi.org/10.3390/ijms25063142.
- Fiske, B.P.; Vander Heiden, M.G. Seeing the Warburg effect in the developing retina. Nat. Cell Biol. 2012, 14, 790–791.
- Winkler, B.S.; Pourcho, R.G.; Starnes, C.; Slocum, J.; Slocum, N. Metabolic mapping in mammalian retina: A biochemical and 3H-2-deoxyglucose autoradiographic study. Exp. Eye Res. 2003, 77, 327–337. https://doi.org/10.1016/s0014-4835(03)00147-7.
- Wang, L.; Kondo, M.; Bill, A. Glucose metabolism in cat outer retina: Effects of light and hyperoxia. Investig. Ophthalmol. Vis. Sci. 1997, 38, 48–55.
- Winkler, B.S. Glycolytic and oxidative metabolism in relation to retinal function. J. Gen. Physiol. 1981, 77, 667–692.
- Kanow, M.A.; Giarmarco, M.M.; Jankowski Connor, S.R.; Kristine, T.; Engel, A.L.; Jianhai, D.; Linton, J.D.; Farnsworth, C.C.; Sloat, S.R.; Austin, R. Biochemical adaptations of the retina and retinal pigment epithelium support a metabolic ecosystem in the vertebrate eye. eLife 2017, 6, e28899.
- Wang, W.; Lee, S.J.; Scott, P.A.; Lu, X.; Emery, D.; Liu, Y.; Ezashi, T.; Roberts, M.R.; Ross, J.W.; Kaplan, H.J. Two-Step Reactivation of Dormant Cones in Retinitis Pigmentosa. Cell Rep. 2016, 15, 372–385.
- Yekai, W.; Allison, G.; Fanyi, Z.; Michelle, Y.; Allison, H.; Elizabeth, G.; Siyan, Z.; Daniel, L.; Jiangjiang, Z.; Jianhai, D. Metabolic signature of the aging eye in mice. Neurobiol. Aging 2018, 71, 223–233.
- Heng, J.S.; Rattner, A.; Stein-O’Brien, G.L.; Winer, B.L.; Jones, B.W.; Vernon, H.J.; Goff, L.A.; Nathans, J. PNAS Plus: Hypoxia tolerance in the Norrin-deficient retina and the chronically hypoxic brain studied at single-cell resolution. Proc. Natl. Acad. Sci. USA 2019, 116, 9103–9114.
- Joyal, J.-S.; Sun, Y.; Gantner, M.L.; Shao, Z.; Evans, L.P.; Saba, N.; Fredrick, T.; Burnim, S.; Kim, J.S.; Patel, G. Retinal lipid and glucose metabolism dictates angiogenesis through the lipid sensor Ffar1. Nat. Med. 2016, 22, 439–445.
- Ng, S.K.; Wood, J.P.; Chidlow, G.; Han, G.; Kittipassorn, T.; Peet, D.J.; Casson, R.J. Cancer-like metabolism of the mammalian retina. Clin. Exp. Ophthalmol. 2015, 43, 367–376.
- Yashodhan, C.; Tedi, B.; David, W.; Eugene, D.; Cepko, C.L. Glycolytic reliance promotes anabolism in photoreceptors. eLife 2017, 6, e25946.
- Young, R.W. The renewal of photoreceptor cell outer segments. J. Cell Biol. 1967, 33, 61–72. https://doi.org/10.1083/jcb.33.1.61.
- Agathocleous, M.; Love, N.K.; Randlett, O.; Harris, J.J.; Liu, J.; Murray, A.J.; Harris, W.A. Metabolic differentiation in the embryonic retina. Nat. Cell Biol. 2012, 14, 859.
- Hsu, S.C.; Molday, R.S. Glucose metabolism in photoreceptor outer segments. Its role in phototransduction and in NADPH-requiring reactions. J. Biol. Chem. 1994, 269, 17954–17959.
- Murray, A.R.; Fliesler, S.J.; Al-Ubaidi, M.R. Rhodopsin: The Functional Significance of Asn-Linked Glycosylation and Other Post-Translational Modifications. Ophthalmic Genet. 2009, 30, 109–120.
- Asano, T.; Katagiri, H.; Takata, K.; Lin, J.L.; Yazaki, Y. The Role of N-Glycosylation of GLUT1 for Glucose Transport Activity. J. Biol. Chem. 1992, 266, 24632–24636.
- Punzo, C.; Xiong, W.; Cepko, C.L. Loss of daylight vision in retinal degeneration: Are oxidative stress and metabolic dysregulation to blame? J. Biol. Chem. 2012, 287, 1642–1648.
- Winkler, B.S.; Arnold, M.J.; Brassell, M.A.; Sliter, D.R. Glucose dependence of glycolysis, hexose monophosphate shunt activity, energy status, and the polyol pathway in retinas isolated from normal (nondiabetic) rats. Investig. Ophthalmol. Vis. Sci. 1997, 38, 62.
- Guy, C.; Matan, C.; Devora, M.O.; Shelly, S.; Ayala, E.; Eyal, B.; Alexey, O. Course of Sodium Iodate–Induced Retinal Degeneration in Albino and Pigmented Mice. Investig. Ophthalmol. Vis. 2017, 58, 2239–2249.
- Swarup, A.; Samuels, I.S.; Bell, B.A.; Han, J.Y.S.; Du, J.; Massenzio, E.; Abel, E.D.; Boesze-Battaglia, K.; Peachey, N.S.; Philp, N.J. Modulating GLUT1 expression in retinal pigment epithelium decreases glucose levels in the retina: Impact on photoreceptors and Müller glial cells. Am. J. Physiol. 2019, 316, C121–C133..
- Hurley, J.B. Retina Metabolism and Metabolism in the Pigmented Epithelium: A Busy Intersection. Annu. Rev. Vis.Sci. 2021, 7, 665-692.
- Punzo, C.; Kornacker, K.; Cepko, C.L. Stimulation of the insulin/mTOR pathway delays cone death in a mouse model of retinitis pigmentosa. Nat. Neurosci. 2009, 12, 44–52.
- Thierry, L.; Najate, A.T.A. Cell Signaling with Extracellular Thioredoxin and Thioredoxin-Like Proteins: Insight into Their Mechanisms of Action. Oxidative Med. Cell. Longev. 2017, 2017, 8475125.
- Léveillard, T.; Philp, N.J.; Sennlaub, F. Is Retinal Metabolic Dysfunction at the Center of the Pathogenesis of Age-related Macular Degeneration? Int. J. Mol.Sci. 2019, 20, 762.
- Teal, C.J.; Ho, M.T.; Huo, L.; Harada, H.; Bahlmann, L.C.; Léveillard, T.; Monnier, P.P.; Ramachandran, A.; Shoichet, M.S. Affinity-controlled release of rod-derived cone viability factor enhances cone photoreceptor survival. Acta Biomater. 2023, 161, 37–49.
- Liang, J.; Yao, F.; Fang, D.; Chen, L.; Zou, Z.; Feng, L.; Zhuang, Y.; Xie, T.; Wei, P.; Li, P.; et al. Hyperoside alleviates photoreceptor degeneration by preventing cell senescence through AMPK-ULK1 signaling. FASEB J. 2023, 37, e23250.
- Xu, L.; Brown, E.E.; Keuthan, C.J.; Gubbi, H.; Grellier, E.K.; Roger, J.; Swaroop, A.; Du, J.; Ash, J.D. AMP-activated-kinase (AMPK) is essential sensor and metabolic regulator of retinal neurons and their integrated metabolism with RPE. bioRxiv 2020, bioRxiv:2020.05.22.109165.
- Koch, S.F.; Duong, J.K.; Hsu, C.W.; Tsai, Y.T.; Lin, C.S.; Wahl-Schott, C.A.; Tsang, S.H. Genetic rescue models refute nonautonomous rod cell death in retinitis pigmentosa. Proc. Natl. Acad. Sci. USA 2017, 114, 5259.
- Han, G.; Wood, J.P.M.; Chidlow, G.; Mammone, T.; Casson, R.J. Mechanisms of neuroprotection by glucose in rat retinal cell cultures subjected to respiratory inhibition. Investig. Ophthalmol. Vis. Sci. 2013, 54, 7567–7577.
- Szczesny, P.J.; Walther, P.; Müller, M. Light damage in rod outer segments: The effects of fixation on ultrastructural alterations. Curr. Eye Res. 1996, 15, 807–814.
- Fan, B.; Zhang, C.; Chi, J.; Liang, Y.; Bao, X.; Cong, Y.; Yu, B.; Li, X.; Li, G.Y. The Molecular Mechanism of Retina Light Injury Focusing on Damage from Short Wavelength Light. Oxid Med. Cell. Longev. 2022, 2022, 8482149. https://doi.org/10.1155/2022/8482149.
- Meschede, I.P.; Ovenden, N.C.; Seabra, M.C.; Futter, C.E.; Votruba, M.; Cheetham, M.E.; Burgoyne, T. Symmetric arrangement of mitochondria:plasma membrane contacts between adjacent photoreceptor cells regulated by Opa1. Proc. Natl. Acad. Sci. USA 2020, 117, 15684–15693. https://doi.org/10.1073/pnas.2000304117.
- Shang, Y.M.; Wang, G.S.; Sliney, D.H.; Yang, C.H.; Lee, L.L. Light-emitting-diode induced retinal damage and its wavelength dependency in vivo. Int. J. Ophthalmol. 2017, 10, 191–202. https://doi.org/10.18240/ijo.2017.02.03.
- Nunnari, J.; Suomalainen, A. Mitochondria: In Sickness and in Health. Cell 2012, 148, 1145–1159.
- Forman, H.J.; Zhang, H. Author Correction: Targeting oxidative stress in disease: Promise and limitations of antioxidant therapy. Nat. Rev. Drug Discov. 2021, 20, 652.
- Scialò, F.; Fernández-Ayala, D.J.; Sanz, A. Role of Mitochondrial Reverse Electron Transport in ROS Signaling: Potential Roles in Health and Disease. Front. Physiol. 2017, 8, 428.
- Jiménez-Loygorri, J.I.; Benítez-Fernández, R.; Viedma-Poyatos, Á.; Zapata-Muñoz, J.; Villarejo-Zori, B.; Gómez-Sintes, R.; Boya, P. Mitophagy in the retina: Viewing mitochondrial homeostasis through a new lens. Prog. Retin. Eye Res. 2023, 96, 101205. https://doi.org/10.1016/j.preteyeres.2023.101205.
- Ratnayake, K.; Payton, J.L.; Meger, M.E.; Godage, N.H.; Karunarathne, A. Blue light-triggered photochemistry and cytotoxicity of retinal. Cell. Signal. 2020, 69, 109547-.
- Shindou, H.; Koso, H.; Sasaki, J.; Nakanishi, H.; Sagara, H.; Nakagawa, K.M.; Takahashi, Y.; Hishikawa, D.; Iizuka-Hishikawa, Y.; Tokumasu, F. Docosahexaenoic acid preserves visual function by maintaining correct disc morphology in retinal photoreceptor cells. J. Biol. Chem. 2017, 292, 12054–12064.
- Organisciak, D.T.; Darrow, R.M.; Barsalou, L.; Kutty, R.K.; Wiggert, B. Susceptibility to Retinal Light Damage in Transgenic Rats with Rhodopsin Mutations. Investig. Ophthalmol. Vis. Sci. 2003, 44, 486–492.
- Zhao, L.; Wang, C.; Song, D.; Li, Y.; Song, Y.; Su, G.; Dunaief, J.L. Systemic administration of the antioxidant/iron chelator α-lipoic acid protects against light-induced photoreceptor degeneration in the mouse retina. Investig. Ophthalmol. Vis. Sci. 2014, 55, 5979–5988. https://doi.org/10.1167/iovs.14-15025.
- Luo, M.M.; Chen, L.; Wang, S.; Zeng, C.; Li, D.Z.; Bi, Y.; Liu, L.Q.; Cai, S.J. The Effect of A2E on the Uptake and Release of Calcium in the Lysosomes and Mitochondria of Human RPE Cells Exposed to Blue Light. J. Ophthalmol. 2021, 2021, 5586659. https://doi.org/10.1155/2021/5586659.
- Li, S.; Ma, H.; Yang, F.; Ding, X. cGMP Signaling in Photoreceptor Degeneration. Int. J. Mol. Sci. 2023, 24, 11200. https://doi.org/10.3390/ijms241311200.
- Chen, E.; Forslind, P.B. Distribution of calcium and sulphur in the blue-light-exposed rat retina. Graef. Arch. Clin. Exp. Ophthalmol. 1995, 233, 163–167.
- Das, S.; Chen, Y.; Yan, J.; Christensen, G.; Belhadj, S.; Tolone, A.; Paquet-Durand, F. The role of cGMP-signalling and calcium-signalling in photoreceptor cell death: Perspectives for therapy development. Pflügers Arch. Eur. J.Physiol. 2021, 473, 1411–1421.
- Maeda, A.; Palczewska, G.; Golczak, M.; Kohno, H.; Palczewski, K. Two-photon microscopy reveals early rod photoreceptor cell damage in light-exposed mutant mice. Proc. Natl. Acad. Sci. USA 2014, 111, E1428.
- Grimm, C.; Wenzel, A.; Hafezi, F.; Yu, S.; Redmond, T.M.; Remé, C.E. Protection of Rpe65-deficient mice identifies rhodopsin as a mediator of light-induced retinal degeneration. Nat. Genet. 2000, 25, 63–66.
- Maeda, A.; Maeda, T.; Golczak, M.; Chou, S.; Desai, A.; Hoppel, C.L.; Matsuyama, S.; Palczewski, K. Involvement of all-trans-retinal in acute light-induced retinopathy of mice. J. Biol. Chem. 2009, 284, 15173–15183.
- Chen, Y.; Okano, K.; Maeda, T.; Chauhan, V.; Golczak, M.; Maeda, A.; Palczewski, K. Mechanism of All-trans-retinal Toxicity with Implications for Stargardt Disease and Age-related Macular Degeneration. J. Biol. Chem. 2012, 287, 5059–5069.
- Chistyakov, D.V.; Baksheeva, V.E.; Tiulina, V.V.; Goriainov, S.V.; Azbukina, N.V.; Gancharova, O.S.; Arifulin, E.A.; Komarov, S.V.; Chistyakov, V.V.; Tikhomirova, N.K. Mechanisms and Treatment of Light-Induced Retinal Degeneration-Associated Inflammation: Insights from Biochemical Profiling of the Aqueous Humor. Int. J. Mol. Sci. 2020, 21, 704.
- Zhang, Y.; Zhao, Z.; Zhao, X.; Xie, H.; Zhang, C.; Sun, X.; Zhang, J. HMGB2 causes photoreceptor death via down-regulating Nrf2/HO-1 and up-regulating NF-κB/NLRP3 signaling pathways in light-induced retinal degeneration model. Free Radic. Biol. Med. 2022, 181, 14–28.
- Rong, R.; Yang, R.; Li, H.; You, M.; Liang, Z.; Zeng, Z.; Zhou, R.; Xia, X.; Ji, D. The roles of mitochondrial dynamics and NLRP3 inflammasomes in the pathogenesis of retinal light damage. Ann. N. Y. Acad. Sci. 2022, 1508, 78–91.
- McAnany, J.J.; Park, J.C. Rod photoreceptor activation and deactivation in early-stage diabetic eye disease. Doc. Ophthalmol. 2023, 146, 229–239. https://doi.org/10.1007/s10633-023-09925-y.
- Li, H.; Liu, X.; Zhong, H.; Fang, J.; Li, X.; Shi, R.; Yu, Q. Research progress on the pathogenesis of diabetic retinopathy. BMC Ophthalmol. 2023, 23, 372. https://doi.org/10.1186/s12886-023-03118-6.
- Berkowitz, B.A.; Kern, T.S.; Bissig, D.; Patel, P.; Bhatia, A.; Kefalov, V.J.; Roberts, R. Systemic Retinaldehyde Treatment Corrects Retinal Oxidative Stress, Rod Dysfunction, and Impaired Visual Performance in Diabetic Mice. Investig. Ophthalmol. Vis. 2015, 56, 6294–6303.
- Du, Y.; Veenstra, A.; Palczewski, K.; Kern, T.S. Photoreceptor cells are major contributors to diabetes-induced oxidative stress and local inflammation in the retina. Proc. Natl. Acad. Sci. USA 2013, 110, 16586–16591.
- Kowluru, R.A.; Kowluru, A.; Mishra, M.; Kumar, B. Oxidative Stress and Epigenetic Modifications in the Pathogenesis of Diabetic Retinopathy. Prog. Retin. Eye Res. 2015, 48, 40–61.
- Lee, S.G.; Lee, C.G.; Yun, I.H.; Hur, D.Y.; Yang, J.W.; Kim, H.W. Effect of lipoic acid on expression of angiogenic factors in diabetic rat retina. Clin. Exp. Ophthalmol. 2012, 40, e47–e57. https://doi.org/10.1111/j.1442-9071.2011.02695.x.
- Liu, H.; Tang, J.; Du, Y.; Saadane, A.; Samuels, I.; Veenstra, A.; Kiser, J.Z.; Palczewski, K.; Kern, T.S. Transducin1, Phototransduction and the Development of Early Diabetic Retinopathy. Investig. Ophthalmol. Vis. Sci. 2019, 60, 1538–1546. https://doi.org/10.1167/iovs.18-26433.
- Liu, H.; Tang, J.; Du, Y.; Saadane, A.; Tonade, D.; Samuels, I.; Veenstra, A.; Palczewski, K.; Kern, T.S. Photoreceptor Cells Influence Retinal Vascular Degeneration in Mouse Models of Retinal Degeneration and Diabetes. Investig. Ophthalmol. Vis. Sci. 2016, 57, 4272–4281. https://doi.org/10.1167/iovs.16-19415.
- de Gooyer, T.E.; Stevenson, K.A.; Humphries, P.; Simpson, D.A.; Gardiner, T.A.; Stitt, A.W. Retinopathy is reduced during experimental diabetes in a mouse model of outer retinal degeneration. Investig. Ophthalmol. Vis. Sci. 2006, 47, 5561–5568. https://doi.org/10.1167/iovs.06-0647.
- Tonade, D.; Liu, H.; Palczewski, K.; Kern, T.S. Photoreceptor cells produce inflammatory products that contribute to retinal vascular permeability in a mouse model of diabetes. Diabetologia 2017, 60, 2111–2120. https://doi.org/10.1007/s00125-017-4381-5.
- Scuderi, S.; D’amico, A.G.; Federico, C.; Saccone, S.; Magro, G.; Bucolo, C.; Drago, F.; D’Agata, V. Different Retinal Expression Patterns of IL-1α, IL-1β, and Their Receptors in a Rat Model of Type 1 STZ-Induced Diabetes. J. Mol. Neurosci. 2015, 56, 431–439. https://doi.org/10.1007/s12031-015-0505-x.
- Sayyad, Z.; Sirohi, K.; Radha, V.; Swarup, G. 661W is a retinal ganglion precursor-like cell line in which glaucoma-associated optineurin mutants induce cell death selectively. Sci. Rep. 2017, 7, 16855. https://doi.org/10.1038/s41598-017-17241-0.
- Yue, T.; Shi, Y.; Luo, S.; Weng, J.; Wu, Y.; Zheng, X. The role of inflammation in immune system of diabetic retinopathy: Molecular mechanisms, pathogenetic role and therapeutic implications. Front. Immunol. 2022, 13, 1055087. https://doi.org/10.3389/fimmu.2022.1055087.
- Inamdar, S.M.; Lankford, C.K.; Baker, S.A. Photoreceptor Ion Channels in Signaling and Disease. Adv. Exp. Med. Biol. 2023, 1415, 269–276. https://doi.org/10.1007/978-3-031-27681-1_39.
- Bensaoula, T.; Ottlecz, A. Biochemical and ultrastructural studies in the neural retina and retinal pigment epithelium of STZ-diabetic rats: Effect of captopril. J. Ocul. Pharmacol. Ther. 2001, 17, 573–586. https://doi.org/10.1089/10807680152729266.
- Kowluru, R.A. Retinal metabolic abnormalities in diabetic mouse: Comparison with diabetic rat. Curr. Eye Res. 2002, 24, 123–128. https://doi.org/10.1076/ceyr.24.2.123.8158.
- Kowluru, R.A. Mitochondria damage in the pathogenesis of diabetic retinopathy and in the metabolic memory associated with its continued progression. Curr. Med. Chem. 2013, 20, 3226–3233. https://doi.org/10.2174/09298673113209990029.
- Berkowitz, B.A.; Gradianu, M.; Bissig, D.; Kern, T.S.; Roberts, R. Retinal ion regulation in a mouse model of diabetic retinopathy: Natural history and the effect of Cu/Zn superoxide dismutase overexpression. Investig. Ophthalmol. Vis. Sci. 2009, 50, 2351–2358. https://doi.org/10.1167/iovs.08-2918.
- Giordano, C.R.; Roberts, R.; Krentz, K.A.; Bissig, D.; Talreja, D.; Kumar, A.; Terlecky, S.R.; Berkowitz, B.A. Catalase therapy corrects oxidative stress-induced pathophysiology in incipient diabetic retinopathy. Investig. Ophthalmol. Vis. Sci. 2015, 56, 3095–3102. https://doi.org/10.1167/iovs.14-16194.
- Saliba, A.; Du, Y.; Liu, H.; Patel, S.; Roberts, R.; Berkowitz, B.A.; Kern, T.S. Photobiomodulation Mitigates Diabetes-Induced Retinopathy by Direct and Indirect Mechanisms: Evidence from Intervention Studies in Pigmented Mice. PLoS ONE 2015, 10, e0139003. https://doi.org/10.1371/journal.pone.0139003.
- Ahmed, J.; Braun, R.D.; Dunn, R., Jr.; Linsenmeier, R.A. Oxygen distribution in the macaque retina. Investig. Ophthalmol. Vis. Sci. 1993, 34, 516–521.
- Arden, G.B.; Sidman, R.L.; Arap, W.; Schlingemann, R.O. Spare the rod and spoil the eye. Br. J. Ophthalmol. 2005, 89, 764–769. https://doi.org/10.1136/bjo.2004.062547.
- Wang, S.; Birol, G.; Budzynski, E.; Flynn, R.; Linsenmeier, R.A. Metabolic Responses to Light in Monkey Photoreceptors. Curr. Eye Res. 2010, 35, 510–518.
- Wang, S.; Liu, Y.; Tan, J.W.; Hu, T.; Zhang, H.F.; Sorenson, C.M.; Smith, J.A.; Sheibani, N. Tunicamycin-induced photoreceptor atrophy precedes degeneration of retinal capillaries with minimal effects on retinal ganglion and pigment epithelium cells. Exp. Eye Res. 2019, 187, 107756. https://doi.org/10.1016/j.exer.2019.107756.
- D’Amico, A.G.; Maugeri, G.; Reitano, R.; Bucolo, C.; Saccone, S.; Drago, F.; D’Agata, V. PACAP Modulates Expression of Hypoxia-Inducible Factors in Streptozotocin-Induced Diabetic Rat Retina. J. Mol. Neurosci. 2015, 57, 501–509. https://doi.org/10.1007/s12031-015-0621-7.
- Liu, W.; Liu, S.; Li, P.; Yao, K. Retinitis Pigmentosa: Progress in Molecular Pathology and Biotherapeutical Strategies. Int. J. Mol. Sci. 2022, 23, 4883. https://doi.org/10.3390/ijms23094883.
- Noel, N.C.L.; Allison, W.T.; MacDonald, I.M.; Hocking, J.C. Zebrafish and inherited photoreceptor disease: Models and insights. Prog. Retin. Eye Res. 2022, 91, 101096. https://doi.org/10.1016/j.preteyeres.2022.101096.
- Yang, C.; Georgiou, M.; Atkinson, R.; Collin, J.; Al-Aama, J.; Nagaraja-Grellscheid, S.; Johnson, C.; Ali, R.; Armstrong, L.; Mozaffari-Jovin, S.; et al. Pre-mRNA Processing Factors and Retinitis Pigmentosa: RNA Splicing and Beyond. Front. Cell Dev. Biol. 2021, 9, 700276. https://doi.org/10.3389/fcell.2021.700276.
- Tabor, S.J.; Yuda, K.; Deck, J.; Gnanaguru, G.; Connor, K.M. Retinal Injury Activates Complement Expression in Müller Cells Leading to Neuroinflammation and Photoreceptor Cell Death. Cells 2023, 12, 1754. https://doi.org/10.3390/cells12131754.
- Fei, Y.; Hughes, T.E. Nuclear trafficking of photoreceptor protein crx: The targeting sequence and pathologic implications. Investig. Ophthalmol. Vis. Sci. 2000, 41, 2849–2856.
- Mitton, K.P.; Swain, P.K.; Chen, S.; Xu, S.; Zack, D.J.; Swaroop, A. The leucine zipper of NRL interacts with the CRX homeodomain. A possible mechanism of transcriptional synergy in rhodopsin regulation. J. Biol. Chem. 2000, 275, 29794–29799. https://doi.org/10.1074/jbc.M003658200.
- Klymenko, V.; González Martínez, O.G.; Zarbin, M.A. Recent Progress in Photoreceptor Cell-Based Therapy for Degenerative Retinal Disease. Stem Cells Transl. Med. 2024, 13, 332–345. https://doi.org/10.1093/stcltm/szae005.
- Yildirim, Z.; Ucgun, N.I.; Yildirim, F. The role of oxidative stress and antioxidants in the pathogenesis of age-related macular degeneration. Clinics 2011, 66, 743–746.
- Eamegdool, S.S.; Sitiwin, E.I.; Cioanca, A.V.; Madigan, M.C. Extracellular matrix and oxidative stress regulate human retinal pigment epithelium growth. Free. Radic. Biol. Med. 2020, 146, 357–371. https://doi.org/10.1016/j.freeradbiomed.2019.11.018.
- Meyer, J.G.; Garcia, T.Y.; Schilling, B.; Gibson, B.W.; Lamba, D.A. Proteome and Secretome Dynamics of Human Retinal Pigment Epithelium in Response to Reactive Oxygen Species. Sci. Rep. 2019, 9, 15440. https://doi.org/10.1038/s41598-019-51777-7.
- Rowan, S.; Jiang, S.; Chang, M.L.; Volkin, J.; Cassalman, C.; Smith, K.M.; Streeter, M.D.; Spiegel, D.A.; Moreira-Neto, C.; Rabbani, N.; et al. A low glycemic diet protects disease-prone Nrf2-deficient mice against age-related macular degeneration. Free Radic. Biol. Med. 2020, 150, 75–86.
- Kowluru, R.A.; Tang, J.; Kern, T.S. Abnormalities of retinal metabolism in diabetes and experimental galactosemia. VII. Effect of long-term administration of antioxidants on the development of retinopathy. Diabetes 2001, 50, 1938–1942.
- Bazan, N.G. Docosanoids and elovanoids from omega-3 fatty acids are pro-homeostatic modulators of inflammatory responses, cell damage and neuroprotection. Mol. Asp. Med. 2018, 64, 18–33.
- Mishra, M.; Kowluru, R.A. Epigenetic Modification of Mitochondrial DNA in the Development of Diabetic Retinopathy. Investig. Ophthalmol. Vis. Sci. 2015, 56, 5133–5142.
- Caruso, S.M.; Ryu, J.; Quinn, P.M.; Tsang, S.H. Precision metabolome reprogramming for imprecision therapeutics in retinitis pigmentosa. J. Clin. Investig. 2020, 130, 3971–3973.