Downloads
Download


This work is licensed under a Creative Commons Attribution 4.0 International License.
Article
Comparison of Thromboelastography, Conventional Coagulation Tests and Clotting Time in Preclinical Investigations of Anticoagulant Drugs in Rodents
Xinrong Yang, Boning Huang, Ping Tang, Liuqing Lin and Baoqin Lin *
The First Affiliated Hospital of Guangzhou University of Chinese Medicine, Guangdong Clinical Research Academy of Chinese Medicine, Guangdong Engineering Research Center of Commercialization of Medical Institution Preparations and Traditional Chinese Medicines, Guangdong Engineering Technology Research Center of Commercialization of Linnan Special Medical Institution Preparations, Guangzhou, 510405; China
* Correspondence: linbaoqin@gzucm.edu.cn
Received: 11 September 2024; Revised: 24 October 2024; Accepted: 28 October 2024; Published: 22 April 2025
Abstract: Background This aimed to compare thromboelastography (TEG), conventional coagulation tests (CCTs) and clotting time (CT) during the detection of coagulation in rodents. Methods The TEG, CCTs and CT were detected in blood samples from the abdominal aorta to assess the coagulation system in rodents administered with anticoagulants. Similarly, the CT test was performed on blood samples from the retro-orbital venous plexus of mice. Subsequently, the sensitivity of CT to detect coagulation changes in mice administered with different anticoagulants was assessed. Finally, factors influencing the clotting time were investigated, including the sources of blood samples, laparotomy, fasting, irrigation, gender, and different technicians. Results TEG failed to detect rivaroxaban-induced changes in the coagulation system of rats. In contrast, APTT, PT, and CT were significantly prolonged following rivaroxaban administration in rats. APTT and PT exhibited higher sensitivity compared to the clotting time. Furthermore, the clotting time of mice was more sensitive relative to that of rats. Moreover, laparotomy, fasting, irrigation, and gender did not influence the clotting time, however, different technicians did. Conclusion In summary, TEG may not be an appropriate method for assessing the impact of drug-induced prolongation of clotting time in rodents. CCTs and CT are effective screening methods for anticoagulant agents. Besides, the CT appears to be ideal for application in the primary screening of anticoagulant drugs in mice, while CCTs are suitable for rats.
Keywords:
thromboelastography conventional coagulation tests capillary tube method clotting time anticoagulants preclinical study1. Introduction
Venous thromboembolism (VTE), including deep vein thrombosis (DVT) and pulmonary embolism (PE), has been recognized as a major diseases with significant burden, affecting nearly 10 million people worldwide per year [1]. VTE refers to pathological venous clots, potentially increasing the risk of surgery, cancer, long-term disability, and hospitalization for acute medical illness. The above factors can increase the risk of VTE by inducing hypercoagulability, stasis, vascular wall damage or dysfunction [2].
Anticoagulant therapy is the mainstay approach for the management of VTE. Heparin and vitamin K antagonists have been shown to inhibit thrombosis [3], but they are primarily used for frequent blood coagulation monitoring and in cases with life-threatening bleeding complication [4]. To overcome the disadvantages of heparin and the vitamin K antagonists, direct oral anticoagulants (DOACs) have been proposed, comprising the factor Xa (FXa) inhibitors rivaroxaban, apixaban, and edoxaban, and the thrombin inhibitor dabigatran [1,5]. Currently, DOACs are recommended as the first-line anticoagulant treatment options for VTE patients, including those with cancer, because they present similar efficacy and superior safety profile, and are easy to administer [1,6]. Therefore, it is imperative to develop tests for quantifying the anticoagulant effects of these drugs and to manage their associated complications. Among the available tests for evaluating hemostasis, the most frequently used in clinical settings are the conventional coagulation tests (CCTs) and thromboelastography (TEG) [7].
The CCTs consist of prothrombin time (PT), activated partial thromboplastin time (APTT), fibrinogen (FIB), and thrombin time (TT) [8]. The advantages of CCTs include being inexpensive with minimal labor requirements. However, these plasma-based tests not only neglect the effects of platelets and red blood cells, but also fail to detect hyperfibrinolysis that is a highly fatal complication of acute hemorrhage [7]. Currently, the use of CCTs to detect trauma-induced coagulopathy is limited because this test takes long time to produce results, cannot completely characterize multiple coagulation abnormalities, and it is poorly associated with clinical results [9].
On the other hand, TEG, which was developed by Hartert in 1948, is a versatile and comprehensive tool [10], capable of measuring specific components of blood coagulation and has been increasingly used in diverse clinical environments such as cardiothoracic surgery [11], liver transplantation [12], trauma [13], sepsis [14], and hemophilia [15]. The use of TEG is based on changes in blood viscosity, and provides an overview of multiple coagulation processes from initial thrombin production to the formation of fibrin strands and fibrinolysis [16]. Unlike CCTs, TEG provides early and more comprehensive description of the global hemostatic function of intravascular coagulation [8,17].
Besides TEG and CCTs, another rapid and accurate parameter for determining blood coagulation is the clotting time [18]. The clotting time was proposed by Sabrazes in 1904 and is usually detected using the capillary tube method [19]. This method can be applied in preclinical studies to test the performance of anticoagulants or active substances.
Although several studies have explored the application of TEG and CCTs in clinical laboratories, the adaptation of such clinical techniques to preclinical animal research lacks clear guidance. Therefore, this study aimed to compare TEG, CCTs, and clotting time in preclinical studies investigating the use of anticoagulant drugs in rodents.
2. Materials and Methods
2.1. Materials
Rivaroxaban was purchased from Bayer (Leverkusen, Germany). Heparin sodium injection was bought from Chengdu Hepatunn Pharmaceutical Co. Ltd. (Chengdu, China). Warfarin was obtained from Shanghai Sine Pharmaceutical Co. Ltd. (Shanghai, China). Thromborel S kit (OUHP29) and Dade Actin Activated Cephaloplastin Reagent kit (B4218-1) were purchased from Siemens Healthcare Diagnostics Products GmbH. Kaolin TEG kit was obtained from the Haemonetics Corporation (Shanghai, China).
2.2. Animals
Male and female Kunming mice weighing 18–22 g (approved No. SCXK 2018-0034) and Sprague-Dawley rats weighing 220–250 g (approved No. SCXK 2019-0047) were purchased from the Experimental Animal Center of Guangzhou University of Chinese Medicine. These rodents were housed in an environment maintained at 23 ± 3 °C and 40–70% humidity with a 12 h light/12 h dark cycle, and provided with water and food ad libitum. Animals were allowed to acclimate for at least 1 week before experimentation. All the experimental procedures were performed in accordance with the Guidelines on the Care and Use of Laboratory Animals issued by the Chinese Council on Animal Research and the Guidelines of Animal Care. The study was approved by the Ethics Committee of Guangzhou University of Chinese Medicine.
2.3. Study Design
Four separate experiments were performed in this study. In experiment 1, 40 rats were randomly divided into 4 groups (n = 10 per group): normal group, rivaroxaban 1 d group (3.125 mg/kg), rivaroxaban 5 d group (3.125 mg/kg), and heparin group (200 U/kg). In addition, 30 mice were randomly divided into 3 groups (n = 10 per group): normal group, rivaroxaban 1 d group (0.045 mg/10 g), and rivaroxaban 5 d group (0.045 mg/10 g). Animals in the normal group and rivaroxaban 5 d group were orally administered with distilled water and rivaroxaban once daily for 5 consecutive days, respectively. Animals in the rivaroxaban 1 d group received a single oral administration of rivaroxaban. Heparin was injected once via tail vein. Rats were fasted for 12 h and then administered with the final treatments. One hour after the final administration, rats were anesthetized with pentobarbital sodium (36 mg/kg, i.p.) and blood was collected from the abdominal aorta. The TEG, APTT, PT and clotting time were evaluated. For mice models, blood was drawn from the retro-orbital venous plexus 1 h after the final administration and used to calculate the clotting time.
In Experiment 2, mice were administered with different anticoagulant drugs to verify whether the clotting time could reflect the anticoagulant effects of these drugs. Forty mice were randomly divided into 4 groups (n = 10 per group): normal group, rivaroxaban group (0.045 mg/10 g, i.g.), warfarin group (0.0048 mg/10 g, i.g.), and heparin group (3.2 U/10 g, i.v.). Distilled water, rivaroxaban, and warfarin were administered once daily for 5 consecutive days, respectively. Heparin was injected once via tail vein. The clotting time was tested 1 h after the final administration.
Based on the results of experiment 1 and 2, we concluded that the clotting time can be used as an indicator to monitor changes in the coagulation system. Therefore, in the subsequent experiments, the factors probably affecting the results of clotting time in rats and mice were examined.
In experiment 3, we examined the variations in clotting time between arterial and venous blood in rats and assessed whether the laparotomy procedure had any impact on clotting time. Laparotomy procedure involved making a 2-cm midline abdominal incision in rats without inducing bleeding. Moreover, the study investigated the effects of fasting, gavage administration, gender, and variations among different technicians on clotting time in mice.
2.4. TEG Detection
Blood samples were collected into vacuum blood collection tubes with 3.2% sodium citrate (citrate/blood, 1/9, v/v) and placed at room temperature for at least 15 min. All samples were run at 37 °C for 2 h. Clot initiation, formation, strength, and stability were measured with the Thrombelastograph Hemostasis Analyzer 5000 series and its corresponding kit (Haemonetics Corporation, Shanghai, China). All TEG parameters were recorded from standard tracings: reaction time (R, min), coagulation time (K, min), angle (α, degrees), maximum amplitude (MA, mm), clot strength (G, dyn/cm2), and lysis at 30 min (LY30, %).
2.5. APTT and PT Detection
Blood samples were collected into vacuum blood collection tubes with 3.2% sodium citrate. The blood was centrifuged at 2000× g for 10 min at 4 °C to obtain plasma, while then the APTT and PT were tested with a coagulation analyzer (CA-7000; Sysmex Corporation, Kobe, Japan) by using Thromborel S kit and Dade Actin Activated Cephaloplastin Reagent kit, respectively.
2.6. Clotting Time Detection
The clotting time was measured using capillary tube method as described previously with minor modifications. Briefly, nonanticoagulated blood was collected from mice retro-orbital venous plexuses or abdominal aortae in rats using a capillary glass tube. The glass tube was placed horizontally and broken every 30 s. The clotting time was measured as the time from when blood filled the glass tube to when thread-like structure of the blood was observed at the break of the glass tube, and it was recorded in seconds.
2.7. Statistical Analysis
Data were expressed as mean ± SD (standard deviation). The differences among groups were compared by one-way ANOVA (analysis of variance) followed by LSD/Dunnett’s T3 test with SPSS 20.0 software (IBM Corporation, Armonk, NY, USA). p < 0.05 was considered statistically significant.
3. Results
3.1. CCTs and CT are Potential Indicators for Monitoring Changes in the Coagulation System
The performance of TEG, CCTs and CT in determining the clotting time was evaluated in rodents using different anticoagulants.
As shown in Figure 1A, data for the heparin group could not be obtained due to the abnormal shape exhibited by the TEG. Furthermore, there were no statistically significant differences in R time, K time, α angle, and MA parameters of TEG among the normal, rivaroxaban 1 d and rivaroxaban 5 d groups in rats (Figure 1B–E).
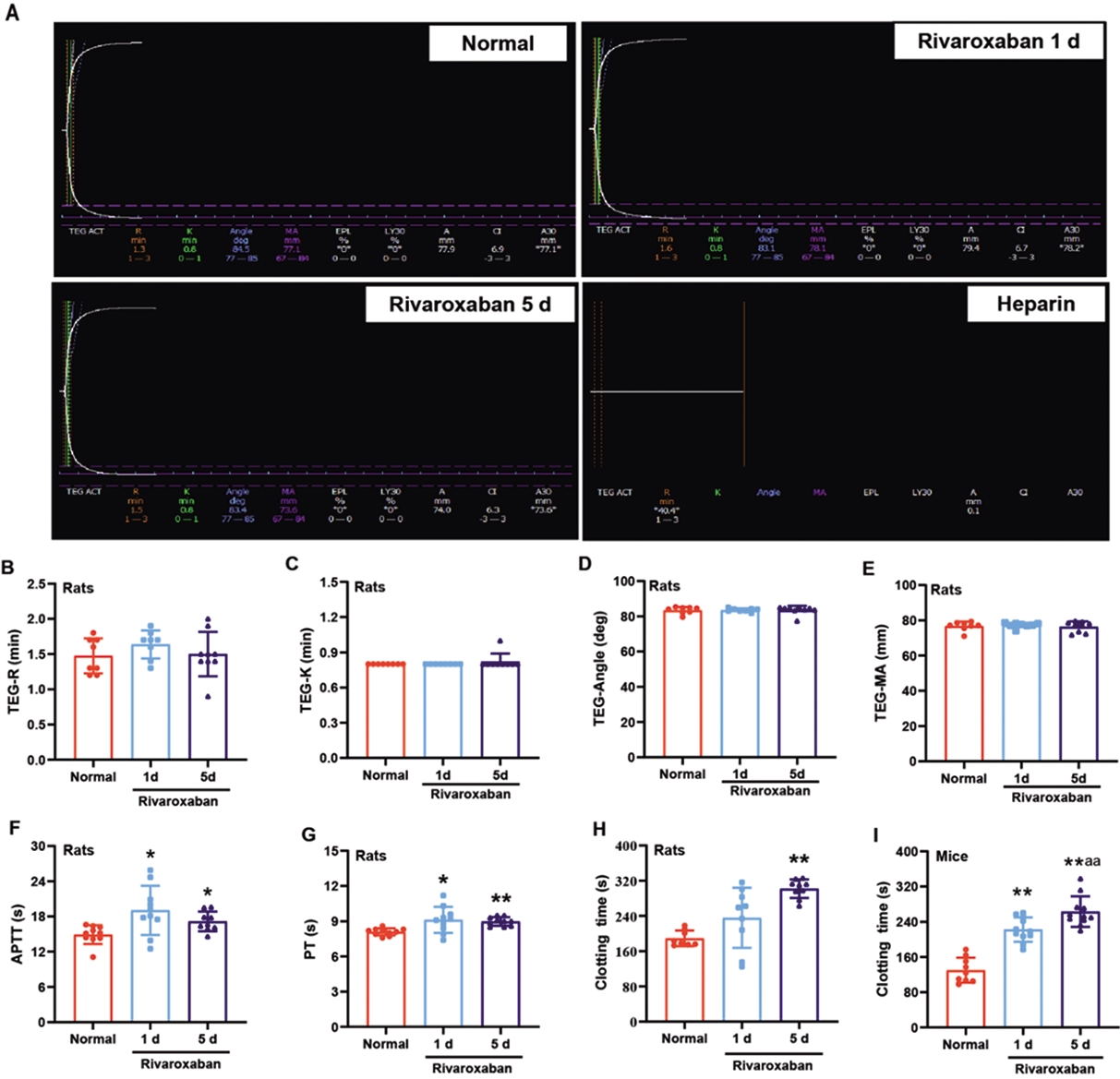
Figure 1. The clotting time was prolonged in rodents treated with rivaroxaban as measured by CCTs and CT. (A) The diagram of TEG in the indicated groups, (B–E) TEG parameters, (F) APTT, (G) PT, (H,I) clotting time. R, reaction time; K, coagulation time; α, angle, rate of clot formation; MA, maximum amplitude; EPL, estimated percentage of lysis; LY30, lysis at 30 min; APTT, activated partial thromboplastin time; PT, prothrombin time. Data are expressed as the mean ± SD, n = 8–10, and each dot represented an individual animal. ** p < 0.01 and * p < 0.05 vs. normal group, and aa p < 0.01 vs. rivaroxaban 1 d group.
The results further showed that administration of rivaroxaban for 1d and 5d increased the APTT and PT in rats compared to the normal group (p < 0.05 or 0.01, Figure 1F,G).
Compared to the control group, rivaroxaban administration for 1 and 5 days significantly prolonged the clotting time in mice (p < 0.01, Figure 1H). Meanwhile, in rats, rivaroxaban achieved significant prolongation of the clotting time (p < 0.01, Figure 1H,I). when administered for 5 days. The clotting time was prolonged by 59.58% in rats and 102.10% in mice following rivaroxaban administration for 5 days. In particular, the clotting time was significantly increased in the rivaroxaban 5 d group in mice compared with the rivaroxaban 1d group (p < 0.01, Figure 1I).
3.2. CT Reflected the Effect of Drug-Induced Prolongation of Activated Clotting Time
The clotting time for rivaroxaban, warfarin, and heparin was significantly higher compared to the normal group (p < 0.001, Figure 2). The longest clotting time was observed in the heparin group, followed by warfarin group and rivaroxaban group.
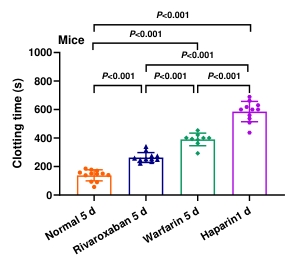
Figure 2. The effects of different anticoagulant drugs on the clotting time in mice. Blood was collected from the retro-orbital venous plexus after 1 d or 5 d of drugs administration. Data are expressed as the mean ± SD, n = 9–12, and each dot represented an individual animal.
3.3. The Investigation of the Factors Affecting Clotting Time in Rats
Compared with the vehicle groups, the clotting time of rivaroxaban groups was significantly longer regardless of whether the blood was harvested from the retro-bulbar venous plexus or the abdominal aorta (p < 0.01, Figure 3A). The coagulation of blood collected from the abdominal aorta was slower than that from the retro-bulbar venous plexus (p < 0.01, Figure 3A).
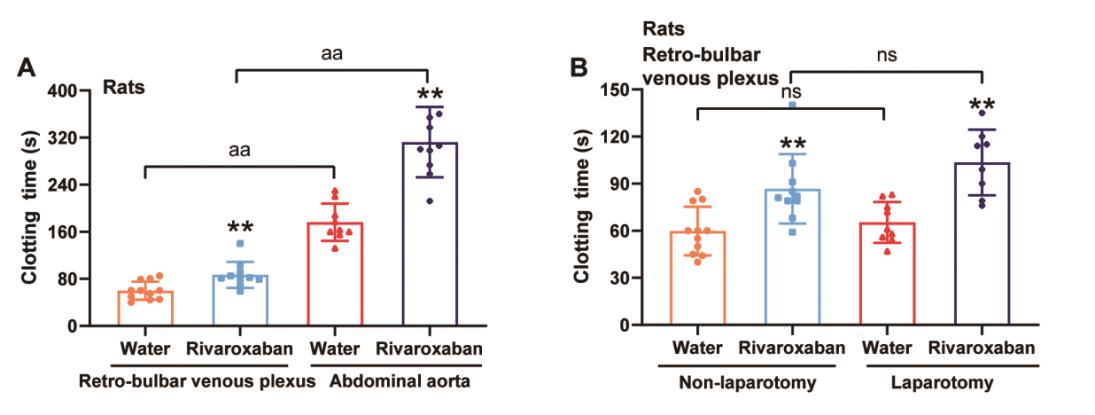
Figure 3. Factors influencing the clotting time in rats. (A) The effect of arterial and venous blood on clotting time in rats. Blood was harvested from the retro-bulbar venous plexus or the abdominal aorta after drugs administration for 5 d. (B) The effect of laparotomy on clotting time in rats. Blood was harvested from the retro-bulbar venous plexus after drugs administration for 5 d. Data were expressed as the mean ± SD, n = 8–11, and each dot represented an individual animal. ** p < 0.01 vs. vehicle group.
Similarly, the clotting time was significantly longer in the rivaroxaban groups, regardless of whether the rats received laparotomy (both p < 0.01 vs. vehicle groups, Figure 3B). However, there was no difference in clotting time between the laparotomy and non-laparotomy groups (Figure 3B).
3.4. Other Potential Factors That May Impact the Clotting Time in Mice
In further analyses, we tested whether fasting, gavage, gender, and different technicians would affect the clotting time measured by the capillary tube method. Notably, there was no difference in clotting time between normal and fasting groups, normal and gavage groups, and male and female groups (Figure 4A–C). However, the clotting time varied significantly among the technicians (p < 0.05, Figure 4D).
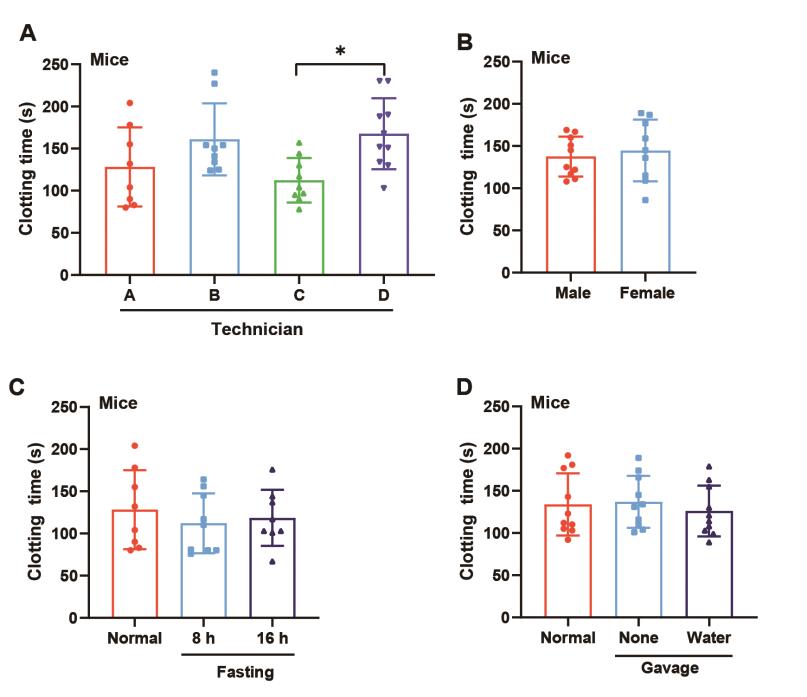
Figure 4. Factors influencing the clotting time in mice. Blood was collected from the retro-orbital venous plexus. The effects of (A) technicians, (B) gender, (C) gavage, and (D) fasting on clotting time in mice. Data are expressed as the mean ± SD, n = 8–11, and each dot represents an individual animal. * p < 0.05 vs. technician C.
4. Discussion
Animal experiments play a crucial role in the discovery and development of drugs for the treatment and prevention of thrombotic diseases. Rodents serve as the primary evaluation models for new FXa inhibitors, such as RPR120844 [20], BAY 59-7939 [21] and YM-60628 [17]. Despite the availability of numerous assays for evaluating the coagulation system in human, these methods are not ideal for animals.
In recent decades, it has been found that TEG assesses coagulation dysfunction and hyperfibrinolysis and is can be an objective assessment for hypercoagulability. It also provides a comprehensive evaluation of viscoelastic properties of whole blood in patients with DVT [22]. In a study conducted in healthy beagles, rivaroxaban administered orally twice daily at 2 mg/kg demonstrated significant anticoagulant effects as measured by PT, APTT, TEG, tissue factor-induced thrombin generation (TG) and anti-factor Xa activity, with peak effects occurring 1.5 to 2 h after administration [23]. In contrast, once-daily oral administration of 1 mg/kg rivaroxaban did not significantly prolong PT, aPTT, or TEG parameters in healthy beagles [24]. In this study, we found that rivaroxaban administered orally once daily at a dose of 3.125 mg/kg did not prolong TEG parameters significantly (Figure 1). This may be due to the hypercoagulability observed in rodents. Researches have revealed that there are great differences between human and animal blood regarding the kinetics of coagulation, clot firmness and clot stability, and suggested that coagulation is faster in all experimental animals [25–28]. Another potential explanation is the low sensitivity of TEG and Rotem tests in detecting the anti-Xa effects of DOACs, which can be assessed using alternative platforms that employ different coagulation activators, such as DRVVT. While some early studies indicated that the TEG kaolin test could effectively monitor the effects of dabigatran, TEG kaolin test and ROTEM tests show poor sensitivity for rivaroxaban [29,30]. Therefore, TEG may not be sensitive enough to assess the impact of rivaroxaban-induced prolongation of clotting time in rodents.
APTT is one of the most commonly used coagulation assays, which is measured as the time from the activation of factor XII to the formation of stable fibrin clots [31]. In the 2021 clinical practice guideline on the management of venous thrombosis, the European Society for Vascular Surgery (ESVS) states that APTT evaluation is necessary for accurate and safe administration [32]. A lower APTT in the first 24 h has been linked to higher incidence of DVT, suggesting that APTT may serve as an adjunct to the VTE risk prediction score [33,34]. Of note, PT allows clinicians to monitor oral anticoagulants that influence the extrinsic coagulation pathway [35]. Rivaroxaban, a direct inhibitor of FXa, prolongs APTT and PT by acting at the convergence point of the extrinsic and intrinsic coagulation pathways [36,37]. Our results showed that rivaroxaban increased clotting time, APTT, and PT in rats, indicating that APTT, PT and clotting time can be used as parameters for anticoagulant drugs in rats.
Moreover, there was no difference in the clotting time between the rivaroxaban 1 d group and the normal group, while the APTT and PT of the rivaroxaban 1 d group were longer than those of the normal group. These results suggest that APTT and PT are more sensitive than clotting time. Additionally, 2 mL of whole blood is usually required to achieve the detections of APTT and PT. Thus, these two parameters are more suitable for mechanistic studies of anticoagulant drugs in rats.
Notably, the clotting time was significantly different between the rivaroxaban 5 d group in mice and the rivaroxaban 1d group. Conversely, no significant difference in the prolongation of clotting time was evident between the rivaroxaban-treated 1 d group and the rivaroxaban-treated 5 d group in rats. These results indicate that mice are more sensitive than rats for evaluating the activity of anticoagulants. Furthermore, we found that the clotting time also reflected the drug effects on coagulation outcomes, which is consistent with the results of other studies [38]. Therefore, the clotting time measured with capillary tube method in mice can serve as a primary screening parameter for anticoagulant drugs owing to its rapid, simple, and economic advantages. An important limitation of this study is that we did not measure drug levels determined by an anti-Xa-specific calibration test to correlate with drug effects on coagulation outcomes.
Data shown in Figure 3A indicate that the clotting time of blood obtained from the retro-bulbar venous plexus was shorter than that from the abdominal aorta in rats. This finding is consistent with other reports, which found that venous samples are more likely to form clots than arterial samples [39,40]. The reasons for these results may include differences in oxygen content with deo-oxygenated blood reported to be more viscous [41]. Laparotomy, fasting, irrigation, and gender did not influence the clotting time. However, the clotting times varied among different technicians. Therefore, to reduce experimental deviation, the measurement of clotting time for specific animal cohorts should be conducted by the same technician.
5. Conclusion
In conclusion, TEG may not be an appropriate method for assessing the effect of drug-induced prolongation of clotting time in rodents. Moreover, APTT, PT and clotting time are effective parameters for testing anticoagulant drugs, with APTT and PT found to be more sensitive than clotting time. Furthermore, clotting time of mice is more sensitive than that of rats. Laparotomy, fasting, irrigation, and gender do not influence the clotting time, while it varies among measurements by different technicians. Therefore, the clotting time is a simple and economical parameter for screening anticoagulant drugs in mice, and CCTs are preferred to study the mechanism of anticoagulant drugs in rats.
Author Contributions: All authors contributed to the study conception and design. X.Y. performed the experiments and wrote the manuscript. B.H. performed the experiments. P.T. contributed to the analysis of data and revising the intellectual content. L.L. provided comments and provided reviews for the final version of the manuscript. B.L. analyzed and interpreted data and revised the manuscript. All authors participated in critically revising the manuscript and approved the final version.
Funding: This work was financially supported by Guangzhou Municipal Science and Technology Project (No. 2024A03J0360), Special Research Project of National Innovation Center of Traditional Chinese medicine (No. 2023ZD01), and GuangDong Engineering Technology Research Center of Lingnan Characteristic Transformation of Medicinal Preparations for Medical Institutes (No. 2023A170).
Institutional Review Board Statement: The study was conducted according to the guidelines of the Declaration of Helsinki, and approved by the Animal Ethics Committee of Guangzhou University of Chinese Medicine (20220301004 and March 1, 2022 of approval)
Informed Consent Statement: Not applicable.
Data Availability Statement: The article materials encompass the novel findings put forth in this investigation, and any additional queries may be directed towards the corresponding authors.
Conflicts of Interest: The authors have declared that there are no conflicting financial or personal interests.
References
- Khan, F.; Tritschler, T.; Kahn, S.R.; et al. Venous thromboembolism. Lancet 2021, 398, 64–77. https://doi.org/10.1016/S0140-6736(20)32658-1.
- Lutsey, P.L.; Zakai, N.A. Epidemiology and prevention of venous thromboembolism. Nat. Rev. Cardiol. 2023, 20, 248–262. https://doi.org/10.1038/s41569-022-00787-6.
- Fredenburgh, J.C.; Weitz, J.I. New anticoagulants: Moving beyond the direct oral anticoagulants. J. Thromb. Haemost. 2021, 19, 20–29. https://doi.org/10.1111/jth.15126.
- Kruger, P.C.; Eikelboom, J.W.; Douketis, J.D.; et al. Deep vein thrombosis: Update on diagnosis and management. Med. J. Aust. 2019, 210, 516–524. https://doi.org/10.5694/mja2.50201.
- Tritschler, T.; Kraaijpoel, N.; Le Gal, G.; et al. Venous Thromboembolism: Advances in Diagnosis and Treatment. JAMA 2018, 320, 1583–1594. https://doi.org/10.1001/jama.2018.14346.
- Di Nisio, M.; Van Es, N.; Büller, H.R. Deep vein thrombosis and pulmonary embolism. Lancet 2016, 388, 3060–3073. https://doi.org/10.1016/S0140-6736(16)30514-1.
- Cohen, T.; Haas, T.; Cushing, M.M. The strengths and weaknesses of viscoelastic testing compared to traditional coagulation testing. Transfusion 2020, 60, S6. https://doi.org/10.1111/trf.16073.
- Mao, C.; Xiong, Y.; Fan, C. Comparison between thromboelastography and conventional coagulation assays in patients with deep vein thrombosis. Clin. Chim. Acta 2021, 520, 208–213. https://doi.org/10.1016/j.cca.2021.06.019.
- Holcomb, J.B.; Minei, K.M.; Scerbo, M.L.; et al. Admission Rapid Thrombelastography Can Replace Conventional Coagulation Tests in the Emergency Department: Experience With 1974 Consecutive Trauma Patients. Ann. Surg. 2012, 256, 476–486. https://doi.org/10.1097/SLA.0b013e3182658180.
- Hartert, H. Blutgerinnungsstudien mit der Thrombelastographie, einem neuen Untersuchungsverfahren. Klin. Wochenschr. 1948, 26, 577–583. https://doi.org/10.1007/BF01697545.
- Redfern, R.E.; Fleming, K.; March, R.L.; et al. Thromboelastography-Directed Transfusion in Cardiac Surgery: Impact on Postoperative Outcomes. Ann. Thorac. Surg. 2019, 107, 1313–1318. https://doi.org/10.1016/j.athoracsur.2019.01.018.
- Pietri, L.D.; Montalti, R.; Bolondi, G.; et al. Intraoperative thromboelastography as a tool to predict postoperative thrombosis during liver transplantation. World J. Transplant. 2020, 10, 345–355. https://doi.org/10.5500/wjt.v10.i11.345.
- Subramanian, M.; Kaplan, L.J.; Cannon, J.W. Thromboelastography-Guided Resuscitation of the Trauma Patient. JAMA Surg. 2019, 154, 1152. https://doi.org/10.1001/jamasurg.2019.3136.
- Muzaffar, S.N.; Azim, A.; Siddiqui, S.S. Thromboelastography for Predicting Disseminated Intravascular Coagulation (DIC) in Sepsis. Shock 2022, 57, 759. https://doi.org/10.1097/SHK.0000000000001929.
- Ramiz, S.; Hartmann, J.; Young, G.; et al. Clinical utility of viscoelastic testing (TEG and ROTEM analyzers) in the management of old and new therapies for hemophilia. Am. J. Hematol. 2019, 94, 249–256. https://doi.org/10.1002/ajh.25319.
- Karon, B.S. Why is everyone so excited about thromboelastrography (TEG)? Clin. Chim. Acta 2014, 436, 143–148. https://doi.org/10.1016/j.cca.2014.05.013.
- Carroll, R.C.; Craft, R.M.; Langdon, R.J.; et al. Early evaluation of acute traumatic coagulopathy by thrombelastography. Transl. Res. 2009, 154, 34–39. https://doi.org/10.1016/j.trsl.2009.04.001.
- Voleisis, A.; Kazys, R.; Voleisiene, B.; et al. Ultrasonic method for monitoring the clotting process during whole blood coagulation. Ultrasonics 2017, 78, 146–151. https://doi.org/10.1016/j.ultras.2017.02.017.
- Biggs, R.; Macmillan, R.L. The Errors of Some Haematological Methods as They Are Used in a Routine Laboratory. J. Clin. Pathol. 1948, 1, 269–287. https://doi.org/10.1136/jcp.1.5.269.
- Heran, C.; Morgan, S.; Kasiewski, C.; et al. Antithrombotic efficacy of RPR208566, a novel factor Xa inhibitor, in a rat model of carotid artery thrombosis. Eur. J. Pharmacol. 2000, 389, 201–207. https://doi.org/10.1016/S0014-2999(99)00902-4.
- Perzborn, E.; Strassburger, J.; Wilmen, A.; et al. In vitro and in vivo studies of the novel antithrombotic agent BAY 59-7939—An oral, direct Factor Xa inhibitor. J. Thromb. Haemost. 2005, 3, 514–521. https://doi.org/10.1111/j.1538-7836.2005.01166.x.
- Brill, J.B.; Badiee, J.; Zander, A.L.; et al. The rate of deep vein thrombosis doubles in trauma patients with hypercoagulable thromboelastography. J. Trauma. Acute Care Surg. 2017, 83, 413–419. https://doi.org/10.1097/TA.0000000000001618.
- Conversy, B.; Blais, M.C.; Dunn, M.; et al. Anticoagulant activity of oral rivaroxaban in healthy dogs. Vet. J. 2017, 223, 5–11. https://doi.org/10.1016/j.tvjl.2017.03.006.
- Evans, L.A.; Tansey, C.; Wiebe, M.; et al. A prospective evaluation of rivaroxaban on haemostatic parameters in apparently healthy dogs. Vet. Med. Sci. 2019, 5, 317–324. https://doi.org/10.1002/vms3.161.
- Gentry, P.A. Comparative aspects of blood coagulation. Vet. J. Lond. Engl. 1997. 2004, 168, 238–251. https://doi.org/10.1016/j.tvjl.2003.09.013.
- Lechner, R.; Helm, M.; Müller, M.; et al. In-vitro study of species-specific coagulation differences in animals and humans using rotational thromboelastometry (ROTEM). BMJ Mil. Health 2019, 165, 356–359. https://doi.org/10.1136/jramc-2018-001092.
- Stettler, G.R.; Moore, E.E.; Moore, H.B.; et al. Thrombelastography indicates limitations of animal models of trauma-induced coagulopathy. J. Surg. Res. 2017, 217, 207–212. https://doi.org/10.1016/j.jss.2017.05.027.
- Wohlauer, M.V.; Moore, E.E.; Harr, J.; et al. A Standardized technique for performing thromboelastography in rodents. Shock 2011, 36, 524–526. https://doi.org/10.1097/SHK.0b013e31822dc518.
- Herrmann, R.; Thom, J.; Wood, A.; et al. Thrombin generation using the calibrated automated thrombinoscope to assess reversibility of dabigatran and rivaroxaban. Thromb. Haemost. 2014, 112, 989–995. https://doi.org/10.1160/TH13-07-0607.
- Dias, J.D.; Norem, K.; Doorneweerd, D.D.; et al. Use of Thromboelastography (TEG) for detection of new oral anticoagulants. Arch. Pathol. Lab. Med. 2015, 139, 665–673. https://doi.org/10.5858/arpa.2014-0170-OA.
- Giani, M.; Russotto, V.; Pozzi, M.; et al. Thromboelastometry, thromboelastography, and conventional tests to assess anticoagulation during extracorporeal support: A Prospective Observational Study. ASAIO J. 2021, 67, 196–200. https://doi.org/10.1097/MAT.0000000000001196.
- Kakkos, S.K.; Gohel, M.; Baekgaard, N.; et al. Editor’s Choice–European Society for Vascular Surgery (ESVS) 2021 Clinical practice guidelines on the management of venous thrombosis. Eur. J. Vasc. Endovasc. Surg. 2021, 61, 9–82. https://doi.org/10.1016/j.ejvs.2020.09.023.
- Hull, R.D.; Raskob, G.E.; Hirsh, J.; et al. Continuous intravenous heparin compared with intermittent subcutaneous heparin in the initial treatment of proximal-vein thrombosis. N. Engl. J. Med. 1986, 315, 1109–1114. https://doi.org/10.1056/NEJM198610303151801.
- Wannamethee, S.G.; Papacosta, O.; Lennon, L.; et al. Haematological variables and risk of future venous thromboembolism in the British Regional Heart Study on men. Combined D-dimer and APTT as a predictive test for thromboembolism? Br. J. Haematol. 2022, 198, 587–594. https://doi.org/10.1111/bjh.18288.
- Lippi, G.; Favaloro, E.J. Laboratory monitoring of warfarin in the era of direct oral anticoagulants. Lancet Haematol. 2015, 2, e223–e224. https://doi.org/10.1016/S2352-3026(15)00075-7.
- Chan, N.; Sobieraj-Teague, M.; Eikelboom, J.W. Direct oral anticoagulants: Evidence and unresolved issues. Lancet Lond. Engl. 2020, 396, 1767–1776. https://doi.org/10.1016/S0140-6736(20)32439-9.
- Perzborn, E.; Roehrig, S.; Straub, A.; et al. Rivaroxaban: A new oral factor Xa inhibitor. Arterioscler. Thromb. Vasc. Biol. 2010, 30, 376–381. https://doi.org/10.1161/ATVBAHA.110.202978.
- Zhou, W.; Abdurahman, A.; Umar, A.; et al. Effects of cydonia oblonga miller extracts on blood hemostasis, coagulation and fibrinolysis in mice, and experimental thrombosis in rats. J. Ethnopharmacol. 2014, 154, 163–169. https://doi.org/10.1016/j.jep.2014.03.056.
- Doering, C.J.; Wagg, C.R.; Caulkett, N.A.; et al. Comparison of arterial and venous whole blood clot initiation, formation, and strength by thromboelastography in anesthetized swine. Blood Coagul. Fibrinolysis. 2014, 25, 20–24. https://doi.org/10.1097/MBC.0b013e328364672a.
- Groves, D.S.; Winegar, D.A.; Fernandez, L.G.; et al. Comparison of coagulation parameters in arterial and venous blood in cardiac surgery measured using the quantra system. J. Cardiothorac. Vasc. Anesth. 2019, 33, 976–984. https://doi.org/10.1053/j.jvca.2018.08.201.
- Schuurman, P.R.; Albrecht, K.W. Intraoperative changes of transcranial Doppler velocity: Relation to arterial oxygen content and whole-blood viscosity. Ultrasound Med. Biol. 1999, 25, 151–154. https://doi.org/10.1016/S0301-5629(98)00135-5.